Silt - A TerraSoil Overview
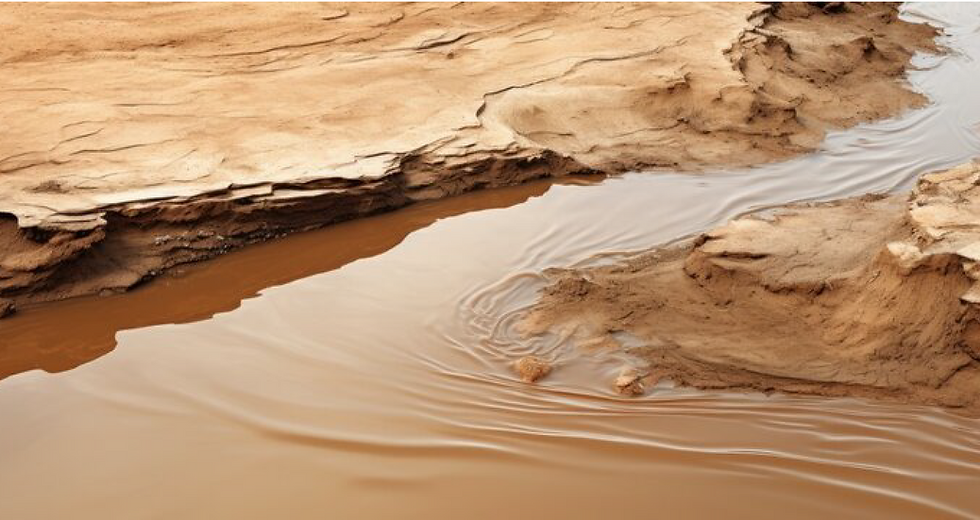
TerraSoil
Aug 3, 2024
Harnessing the Benefits of Silt in Agriculture
Understanding Silt
Silt is a granular material composed of fine mineral particles, ranging in size from 0.002 to 0.05 mm in diameter. It falls between the coarser sand particles and the finer clay particles in terms of particle size. Silt particles are often derived from the weathering and erosion of rocks and minerals, carried by wind, water, or glaciers to deposit in various landscapes.
Formation of Silt
Silt is formed through the mechanical and chemical weathering of rocks and minerals over geological time scales. As rocks break down into smaller fragments through processes such as abrasion, erosion, and dissolution, silt-sized particles are produced and transported by natural agents such as water, wind, and ice.
Silt Types, Composition, Physical Characteristics, and Origin
Silt Type | Composition | Physical Characteristics | Origin | Nutrient Profile |
Aeolian Silt | Quartz, Feldspar, Mica | Fine-grained, low porosity | Wind-blown deposits | N: 0.01-0.05%, P: 0.01-0.03%, K: 0.5-1%, Fe: 1-2%, Mn: 0.1-0.2% |
Alluvial Silt | Quartz, Feldspar, Clay | Moderate texture, high fertility | River and stream deposits | N: 0.1-0.2%, P: 0.05-0.1%, K: 1-2%, Fe: 2-4%, Mn: 0.2-0.5%, Zn: 0.1-0.2% |
Glacial Silt | Quartz, Feldspar, Rock Flour | Smooth texture, high mineral content | Glacial deposits | N: 0.01-0.05%, P: 0.01-0.03%, K: 0.5-1%, Fe: 1-3%, Mn: 0.1-0.2% |
Lacustrine Silt | Clay minerals, Organic matter | Fine texture, high moisture retention | Lake bed deposits | N: 0.1-0.2%, P: 0.05-0.1%, K: 1-1.5%, Fe: 2-3%, Mn: 0.2-0.4%, Zn: 0.1-0.15% |
Marine Silt | Clay, Shell fragments | Fine texture, saline | Coastal and marine deposits | N: 0.05-0.1%, P: 0.02-0.05%, K: 1-1.5%, Fe: 1-2%, Mn: 0.1-0.3% |
Volcanic Silt | Volcanic ash, Pumice | Light, porous, mineral-rich | Volcanic eruptions | N: 0.1-0.2%, P: 0.1-0.15%, K: 2-4%, Fe: 2-5%, Mn: 0.5-1%, Zn: 0.2-0.3% |
Colluvial Silt | Mixed minerals, Organic matter | Varied texture, high fertility | Hillslope deposits | N: 0.1-0.2%, P: 0.05-0.1%, K: 1-2%, Fe: 2-4%, Mn: 0.2-0.5%, Zn: 0.1-0.2% |
Fluvial Silt | Quartz, Clay, Organic matter | Smooth texture, high fertility | Floodplain deposits | N: 0.1-0.2%, P: 0.05-0.1%, K: 1.5-2.5%, Fe: 2-4%, Mn: 0.2-0.4%, Zn: 0.1-0.15% |
Desert Silt | Quartz, Gypsum | Fine, dry, low organic matter | Arid and semi-arid regions | N: 0.01-0.05%, P: 0.01-0.03%, K: 0.5-1%, Fe: 1-2%, Mn: 0.05-0.1% |
Tidal Silt | Clay, Organic matter | Fine, compact, saline | Tidal flats and deltas | N: 0.05-0.1%, P: 0.02-0.04%, K: 1-1.5%, Fe: 1-2%, Mn: 0.1-0.3% |
Loess Silt | Quartz, Feldspar, Calcite | Fine, homogeneous, porous | Wind-blown deposits | N: 0.01-0.05%, P: 0.01-0.03%, K: 0.5-1%, Fe: 1-3%, Mn: 0.1-0.2% |
Estuarine Silt | Clay, Shell fragments | Fine, anoxic, organic-rich | Estuaries | N: 0.1-0.2%, P: 0.05-0.1%, K: 1-2%, Fe: 1-2%, Mn: 0.2-0.4% |
Peat Silt | Organic matter, Peat | Spongy, high organic content | Bog and marsh deposits | N: 0.5-1.5%, P: 0.2-0.4%, K: 0.5-1%, Fe: 1-3%, Mn: 0.2-0.3% |
Biogenic Silt | Organic matter, Carbonates | Fine, organic-rich | Marine and lake deposits | N: 0.1-0.3%, P: 0.05-0.2%, K: 0.5-1%, Fe: 1-2%, Mn: 0.1-0.3% |
Benefits of Silt in Soil
Soil Structure Improvement: Silt particles contribute to soil aggregation and stability, enhancing soil structure and tilth.
Water Retention: Silt soils have good water retention capacity, holding moisture for plant uptake during dry periods.
Nutrient Availability: Silt soils possess a moderate nutrient-holding capacity, providing a reservoir of essential nutrients for plant growth.
Aeration: Silt soils promote soil aeration and root penetration, facilitating gas exchange and enhancing root development.
Negatives of Silt in Soil
Erosion: Silt particles are susceptible to erosion by wind and water, leading to soil degradation and loss of fertility.
Compaction: Silt soils can become compacted under heavy machinery or foot traffic, reducing porosity and inhibiting root growth.
Drainage Issues: Excessive silt content can lead to poor drainage and waterlogging, creating anaerobic conditions detrimental to plant growth.
Soil Crusting: Silt soils are prone to surface crusting, hindering seed germination and seedling emergence.
Optimum Level of Silt in Soil
The optimum level of silt in soil depends on factors such as soil texture, climate, crop type, and management practices. A balanced soil texture, containing a mixture of sand, silt, and clay particles, promotes optimal soil structure and fertility.
Benefits of Adding Silt to Compost
Incorporating silt into compost can improve its texture, water-holding capacity, and nutrient content. Silt particles act as a bulking agent, enhancing compost structure and reducing compaction. Additionally, silt-amended compost provides a source of organic matter and essential nutrients for plant growth.
Dangers of Using Silt and Appropriate PPE
Handling silt can pose health risks due to inhalation of fine particles and skin irritation. Appropriate personal protective equipment (PPE), including dust masks, gloves, and protective clothing, should be worn when working with silt to minimize exposure and ensure safety.
Sustainability of Using Silt
The sustainable use of silt in agriculture relies on responsible management practices that optimize its beneficial properties while mitigating potential drawbacks. Incorporating silt into soil conservation practices, such as cover cropping, contour farming, and erosion control measures, can enhance soil health and minimize environmental impact.
Conclusion
Silt, the gentle giant of fertile soils, embodies the delicate balance between geological forces and ecological harmony. From its humble origins in the Earth's crust to its profound impact on crop productivity and ecosystem resilience, silt remains a cornerstone of sustainable farming practices. Let us honor the silent stewardship of silt and cultivate a future where soil fertility flourishes in harmony with nature's wisdom.
References:
Hillel, D. (2004). Introduction to environmental soil physics. Elsevier Academic Press.
Lal, R. (2015). Soil and water conservation. Springer.
Govers, G., Poesen, J., & Strauss, P. (2002). Soil erosion in Europe: Major processes, causes and consequences. Soil and Tillage Research, 63(1-2), 437-451.
Wang, G., & Feng, S. (2013). Climate change and its impact on soil erosion by water and tillage in the semiarid loess hilly area of China. The Scientific World Journal, 2013.
Lal, R. (2016). Soil health and climate change: Soil biota, soil health and global warming. Springer.
Brady, N. C., & Weil, R. R. (2008). The Nature and Properties of Soils. Pearson Prentice Hall.
Penn, C. J., & Camberato, J. J. (2019). A critical review on soil chemical processes that control how soil pH affects phosphorus availability to plants. Agriculture, 9(6), 120.
SSSA (Soil Science Society of America). (2020). Glossary of Soil Science Terms. Soil Science Society of America.
Lehmann, J., & Joseph, S. (2009). Biochar for environmental management: Science, technology, and implementation. Earthscan.
Edwards, C. A., Arancon, N. Q., & Sherman, R. (2010). Vermiculture technology: Earthworms, organic wastes, and environmental management. CRC Press.
Montgomery, D. R. (2007). Dirt: The erosion of civilizations. University of California Press.
Bhatia, A. (2019). Organic Farming and Climate Change. Springer.
Gosling, P., & Shepherd, M. (2005). Long-term changes in soil fertility in organic arable farming systems in England, with particular reference to phosphorus and potassium. Agriculture, Ecosystems & Environment, 105(1-2), 425-432.